Keywords
Computer Science and Digital Science
- A6.1.1. Continuous Modeling (PDE, ODE)
- A6.2.1. Numerical analysis of PDE and ODE
- A6.2.7. High performance computing
- A6.5. Mathematical modeling for physical sciences
- A6.5.2. Fluid mechanics
Other Research Topics and Application Domains
- B4. Energy
- B4.2. Nuclear Energy Production
- B5.2.1. Road vehicles
- B5.2.3. Aviation
- B5.2.4. Aerospace
1 Team members, visitors, external collaborators
Research Scientists
- Rémi Manceau [Team leader, CNRS, Senior Researcher, HDR]
- Pascal Bruel [CNRS, Researcher, HDR]
- Vincent Perrier [Inria, Researcher]
Faculty Member
- Jonathan Jung [Univ de Pau et des pays de l'Adour, Associate Professor]
Post-Doctoral Fellows
- Syed Jameel [Univ de Pau et des pays de l'Adour, from Dec 2020]
- Franck Mastrippolito [CNRS, until Jan 2020]
- Sangeeth Simon [Inria, from Jul 2020]
PhD Students
- Al Hassan Afailal [IFPEN, until Sep 2020]
- Puneeth Bikkanahally Muni Reddy [Univ de Pau et des pays de l'Adour]
- Vladimir Duffal [EDF]
- Alexis Ferré [CEA-Liten, from Nov 2020 until Oct 2023]
- Syed Jameel [Groupe PSA, until Jan 2020]
- Gaëtan Mangeon [EDF, until Jan 2020]
- Mahitosh Mehta [Univ de Pau et des pays de l'Adour, from Nov 2020]
- Gustave Sporschill [Dassault Aviation]
Interns and Apprentices
- Jairo Cuervo Lopez [Inria, from May 2020 until Oct 2020]
Administrative Assistant
- Sylvie Embolla [Inria]
Visiting Scientist
- Mariovane Sabino Donini [Instituto Nacional de Pesquisas Espaciais - Brésil, until Aug 2020]
2 Overall objectives
This interdisciplinary project brings together researchers coming from different horizons and backgrounds (applied mathematics and fluid mechanics), who gradually elaborated a common vision of what should be the simulation tools for fluid dynamics of tomorrow. Our applications will be focused on wall bounded turbulent flows, featuring complex phenomena such as aeroacoustics, hydrodynamic instabilities, phase change processes, complex walls, buoyancy or localized relaminarization. Because such flows are exhibiting a multiplicity of time and length scales of fluctuations resulting from complex interactions, their simulation is extremely challenging. Even if various methods of simulation (DNS 1) and turbulence modeling(RANS 2, LES 3, hybrid RANS-LES) are available and have been significantly improved over time, none of them does satisfy all the needs encountered in industrial and environmental configurations. We consider that all these methods will be useful in the future in different situations or regions of the flow, if combined in the same simulation in order to benefit from their respective advantages wherever relevant, while mutually compensating their known limitations. It will thus lead to a description of turbulence at widely varying scales in the computational domain, hence the name multi-scale simulations. For example, the RANS mode may extend throughout regions where turbulence is sufficiently close to equilibrium, leaving to LES or DNS the handling of regions where large-scale coherent structures are present. However, a considerable body of work is required to:
- Establish the behavior of the different types of turbulence modeling approaches when combined with high order discretization methods.
- Elaborate relevant and robust switching criteria between models, similar to error assessments used in automatic mesh refinement, but based on the physics of the flow, in order to adapt on the fly the scale of resolution from one extreme of the spectrum to another (say from the Kolmogorov scale to the geometrical scale, i.e., from DNS to RANS).
- Ensure a high level of accuracy and robustness of the resulting simulation tool to address a large range of flow configurations, i.e., from a generic lab-scale geometry for validation to practical systems of interest of our industrial partners.
But the best agile modeling and high-order discretization methods are useless without the recourse to high performance computing (HPC) to bring the simulation time down to values compatible with the requirement of the end users. Therefore, a significant part of our activity will be devoted to the proper handling of the constantly evolving supercomputer architectures. But even the best ever simulation library is useless if it is not disseminated and increasingly used by the CFD community as well as our industrial partners. In that respect, the significant success of the low-order finite volume simulation suite OpenFOAM 4 or the more recently proposed SU2 5 from Stanford are considered as examples of quite successful dissemination stories that could be, if not followed, but at least considered as a source of inspiration. Our natural inclination though will be to promote the use of the library in direction of our present and future industrial and academic partners, with a special interest on the SMEs active in the highly competitive and strategic economical sectors of energy production and aerospace propulsion. Indeed, these sectors are experiencing a revolution of the entire design process especially for complex parts with an intimate mix between simulations and additive manufacturing (3D printing) processes in the early stages of the design process. For large companies, such as General Electric or Safran (co-developing the CFM Leap-1 engines with 3D printed fuel nozzles), as well as medium-size companies such as Aerojet Rocketdyne, this is a unique opportunity to reduce the duration and hence the cost of development of their systems, while preserving if not strengthening their capability of designing innovative components that cannot be produced by classical manufacturing processes. On the other hand, for the small companies of this sector, this may have a rather detrimental effect on their competitiveness since their capability of mastering both these new manufacturing processes and advanced simulation approaches is far more limited. Thus, through our sustained direct (EDF, Turbomeca, PSA group, AD Industrie, Dassault Aviation) or indirect (European programs: WALLTURB, KIAI, IMPACT-AE, SOPRANO; ANR program MONACO_2025) partnership with different companies, we are able to identify relevant generic configurations, from our point of view of scientists, to serve as support for the development of our approach. This methodological choice was motivated by the desire to lead an as efficient as possible transfer activity, while maintaining a clear distinction between what falls within our field of competence of researchers and what is related to the development of their products by our industrial partners. The long-term objective of this project is to develop, validate, promote and transfer an original and effective approach for modeling and simulating generic flows representative of flow configurations encountered in the field of energy production and aeronautical/automotive propulsion. Our approach will be combining mesh (h) + turbulence model (m) + discretization order (p) agility. This will be achieved by:
- Contributing to the development of new turbulence models.
- Improving high order numerical methods, and increasing their efficiency in the constantly evolving High Performance Computing context.
- Developing experimental tools.
Concerning applications, our objective are :
- To reinforce the long term existing partnership with industrial groups active in the sector of energy production and aeronautical/automotive propulsion, and the other European partners involved in the same European projects as we are.
- To consolidate and develop partnership with SMEs operating in the aeronautical sector.
3 Research program
3.1 The scientific context
3.1.1 Computational fluid mechanics: modeling or not before discretizing ?
A typical continuous solution of the Navier-Stokes equations at sufficiently large values of the Reynolds number is governed by a wide spectrum of temporal and spatial scales closely connected with the turbulent nature of the flow. The term deterministic chaos employed by Frisch in his enlightening book 39 is certainly conveying most adequately the difficulty in analyzing and simulating this kind of flows. The broadness of the turbulence spectrum is directly controlled by the Reynolds number defined as the ratio between the inertial forces and the viscous forces. This number is not only useful to determine the transition from a laminar to a turbulent flow regime, it also indicates the range of scales of fluctuations that are present in the flow under consideration. Typically, for the velocity field and far from solid walls, the ratio between the largest scale (the integral length scale) and the smallest one (Kolmogorov scale) is proportional to per dimension, where is the turbulent Reynolds number, based on the length and velocity scales of the largest turbulent eddies. In addition, for internal flows, viscous effects near the solid walls yield a scaling proportional to per dimension, where is the friction Reynolds number. The smallest scales play a crucial role in the dynamics of the largest ones, which implies that an accurate framework for the computation of turbulent flows must take into account all the scales, which can lead to unrealistic computational costs in real-world applications. Thus, the usual practice to deal with turbulent flows is to choose between an a priori modeling (in most situations) or not (low Re number and rather simple configurations) before proceeding to the discretization step, followed by the simulation itself. If a modeling phase is on the agenda, then one has to choose again among the above-mentioned variety of approaches. The different simulation options and their date of availability for high-Reynolds-number applications are illustrated in Fig. 1: simulation of turbulent flows can be achieved either by directly solving the Navier-Stokes equations (DNS) or by first applying to the equations a statistical averaging (RANS), a spatial filtering (LES), or a combination of these two operators (hybrid RANS/LES). The new terms brought about by the operator have to be modeled. From a computational point of view, the RANS approach is the least demanding, which explains why historically it has been the workhorse in both the academic and the industrial sectors, and it remains the standard approach nowadays for industrial design, except for very specific applications. It has permitted quite a substantial progress in the understanding of various phenomena such as turbulent combustion or heat transfer. Its inherent inability to provide a time-dependent information has led to promote in the last decade the recourse to either LES or DNS to supplement if not replace RANS. By simulating the large scale structures while modeling the smallest ones, assumed more isotropic, LES proved to be quite a breakthrough to fully take advantage of the increasing power of computers to study complex flow configurations. At the same time, DNS was gradually applied to geometries of increasing complexity (channel flows with values of multiplied by 45 during the last 30 years, jets, turbulent premixed flames, among many others), and proved to be a formidable tool to (i) improve our knowledge on turbulent flows and (ii) test (i.e., validate or invalidate) and improve the modeling hypotheses inherently associated to the RANS and LES approaches. From a numerical point of view, due to the steady nature of the RANS equations, numerical accuracy is generally not ensured via the use of high-order schemes, but rather on careful grid convergence studies. In contrast, the high computational cost of LES or DNS makes necessary the use of highly-accurate numerical schemes in order to optimize the use of computational resources.
To the noticeable exception of the hybrid RANS-LES modeling, which is not yet accepted as a reliable tool for industrial design, as mentioned in the preamble of the Go4hybrid European program 6, a turbulence model represents turbulent mechanisms in the same way in the whole flow. Thus, depending on its intrinsic strengths and weaknesses, accuracy will be a rather volatile quantity, strongly dependent on the flow configuration. For instance, RANS is perfectly suited to attached boundary layers, but exhibits severe limitations in massively-separated flow regions. Therefore, the turbulence modeling and industrial design communities waver between the desire to continue to rely on the RANS approach, which is unrivaled in terms of computational cost, but is still not able to accurately represent all the complex phenomena; and the temptation to switch to LES, which outperforms RANS in many situations, but is prohibitively expensive in high-Reynolds number wall-bounded flows. In order to account for the limitations of the two approaches and to combine them for significantly improving the overall performance of the models, the hybrid RANS-LES approach has emerged during the last two decades as a viable, intermediate way, and we are definitely inscribing our project in this innovative field of research, with an original approach though, based on temporal filtering (Hybrid temporal LES, HTLES) rather than spatial filtering, and a systematic and progressive validation process against experimental data produced by the team.
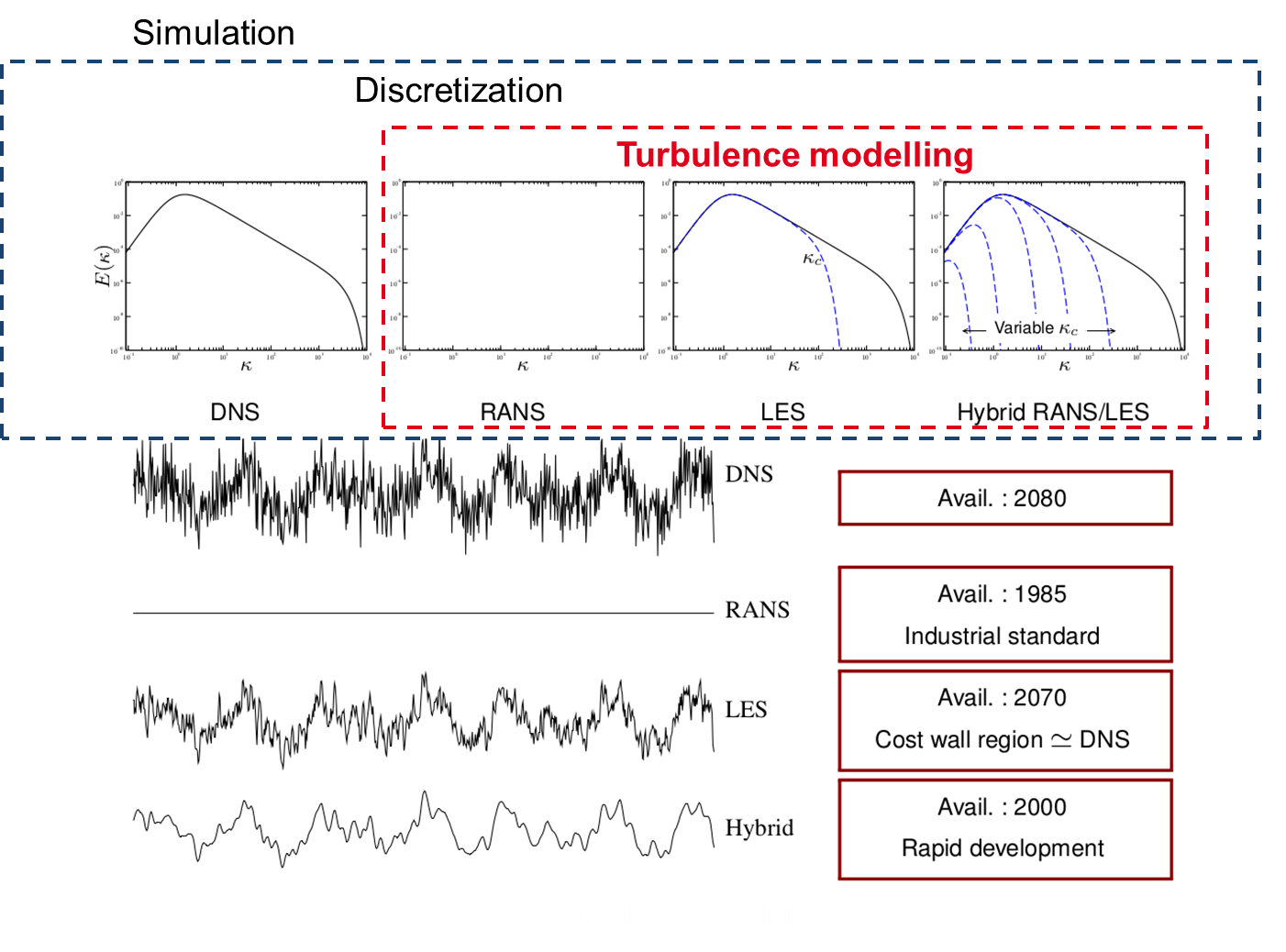
3.1.2 Computational fluid mechanics: high order discretization on unstructured meshes and efficient methods of solution
All the methods considered in the project are mesh-based methods: the computational domain is divided into cells, that have an elementary shape: triangles and quadrangles in two dimensions, and tetrahedra, hexahedra, pyramids, and prisms in three dimensions. If the cells are only regular hexahedra, the mesh is said to be structured. Otherwise, it is said to be unstructured. If the mesh is composed of more than one sort of elementary shape, the mesh is said to be hybrid. In the project, the numerical strategy is based on discontinuous Galerkin methods. These methods were introduced by Reed and Hill 55 and first studied by Lesaint and Raviart 49. The extension to the Euler system with explicit time integration was mainly led by Shu, Cockburn and their collaborators. The steps of time integration and slope limiting were similar to high-order ENO schemes, whereas specific constraints given by the finite-element nature of the scheme were gradually solved for scalar conservation laws 34, 32, one dimensional systems 31, multidimensional scalar conservation laws 30, and multidimensional systems 33. For the same system, we can also cite the work of 38, 45, which is slightly different: the stabilization is made by adding a nonlinear term, and the time integration is implicit. In contrast to continuous Galerkin methods, the discretization of diffusive operators is not straightforward. This is due to the discontinuous approximation space, which does not fit well with the space function in which the diffusive system is well posed. A first stabilization was proposed by Arnold 22. The first application of discontinuous Galerkin methods to Navier-Stokes equations was proposed in 27 by mean of a mixed formulation. Actually, this first attempt led to a non-compact computational stencil, and was later proved to be unstable. A compactness improvement was made in 28, which was later analyzed, and proved to be stable in a more unified framework 23. The combination with the RANS model was made in 26. As far as Navier-Stokes equations are concerned, we can also cite the work of 41, in which the stabilization is closer to the one of 23, the work of 50 on local time stepping, or the first use of discontinuous Galerkin methods for direct numerical simulation of a turbulent channel flow done in 35. Discontinuous Galerkin methods became very popular because:
- They can be developed for any order of approximation.
- The computational stencil of one given cell is limited to the cells with which it has a common face. This stencil does not depend on the order of approximation. This is a pro, compared for example with high-order finite volumes, for which the number of neighbors required increases with the order of approximation.
- They can be developed for any kind of mesh, structured, unstructured, but also for aggregated grids 25. This is a pro compared not only with finite-difference schemes, which can be developed only on structured meshes, but also compared with continuous finite-element methods, for which the definition of the approximation basis is not clear on aggregated elements.
- -adaptivity is easier than with continuous finite elements, because neighboring elements having a different order are only weakly coupled.
- Upwinding is as natural as for finite volumes methods, which is a benefit for hyperbolic problems.
- As the formulation is weak, boundary conditions are naturally weakly formulated. This is a benefit compared with strong formulations, for example point centered formulation when a point is at the intersection of two kinds of boundary conditions.
For concluding this section, there already exists numerical schemes based on the discontinuous Galerkin method, which proved to be efficient for computing compressible viscous flows. Nevertheless, there remain many things to be improved, which include: efficient shock capturing methods for supersonic flows, high-order discretization of curved boundaries, low-Mach-number behavior of these schemes and combination with second-moment RANS closures. Another aspect that deserves attention is the computational cost of discontinuous Galerkin methods, due to the accurate representation of the solution, calling for a particular care of implementation for being efficient. We believe that this cost can be balanced by the strong memory locality of the method, which is an asset for porting on emerging many-core architectures.
3.1.3 Experimental fluid mechanics: a relevant tool for physical modeling and simulation development
With the considerable and constant development of computer performance, many people were thinking at the turn of the 21st century that in the short term, CFD would replace experiments, considered as too costly and not flexible enough. Simply flipping through scientific journals such as Journal of Fluid Mechanics, Combustion and Flame, Physics of Fluids or Journal of Computational Physics or through websites such that of Ercoftac 7 is sufficient to convince oneself that the recourse to experiments to provide either a quantitative description of complex phenomena or reference values for the assessment of the predictive capabilities of models and simulations is still necessary. The major change that can be noted though concerns the content of the interaction between experiments and CFD (understood in the broad sense). Indeed, LES or DNS assessment calls for the experimental determination of temporal and spatial turbulent scales, as well as time-resolved measurements and determination of single or multi-point statistical properties of the velocity field. Thus, the team methodology incorporates from the very beginning an experimental component that is operated in strong interaction with the modeling and simulation activities.
3.2 Research directions
3.2.1 Boundary conditions
Generating synthetic turbulence
A crucial point for any multi-scale simulation able to locally switch (in space or time) from a coarse to a fine level of description of turbulence, is the enrichment of the solution by fluctuations as physically meaningful as possible. Basically, this issue is an extension of the problem of the generation of realistic inlet boundary conditions in DNS or LES of subsonic turbulent flows. In that respect, the method of anisotropic linear forcing (ALF) we have developed in collaboration with EDF proved very encouraging, by its efficiency, its generality and simplicity of implementation. So, it seems natural, on the one hand, to extend this approach to the compressible framework and to implement it in AeroSol. On the other hand, we shall concentrate (in cooperation with EDF R&D in Chatou in the framework of a the CIFRE PhD of V. Duffal) on the theoretical link between the local variations of the scale of description of turbulence (e.g. a sudden variations in the size of the time filter) and the intensity of the ALF forcing, transiently applied to promote the development of missing fluctuating scales.
Stable and non reflecting boundary conditions
In aerodynamics, and especially for subsonic computations, handling inlet and outlet boundary conditions is a difficult issue. A significant amount of work has already been performed for second-order schemes for Navier-Stokes equations, see 54, 60 and the huge number of papers citing it. On the one hand, we believe that decisive improvements are necessary for higher-order schemes: indeed, the less dissipative the scheme is, the worse impact have the spurious reflections. For this purpose, we will first concentrate on the linearized Navier-Stokes system, and analyze the way to impose boundary conditions in a discontinuous Galerkin framework with a similar approach as in 40. We will also try to extend the work of 61, which deals with Euler equations, to the Navier-Stokes equations.
3.2.2 Turbulence models and model agility
Extension of zero-Mach models to the compressible system
We shall develop in parallel our multi-scale turbulence modeling and the related adaptive numerical methods of AeroSol. Without prejudice to methods that will be on the podium in the future, a first step in this direction will be to extend to a compressible framework the continuous temporal hybrid RANS/LES method we have developed up to now in a Mach zero context.
Study of wall flows with and without mass or heat transfer at the wall: determination and validation of relevant criteria for hybrid turbulence models
In the targeted application domains, turbulence/wall interactions and heat transfer at the fluid-solid interface are physical phenomena whose numerical prediction is at the heart of the concerns of our industrial partners. For instance, for a jet engine manufacturer, being able to properly design the configuration of the cooling of the walls of its engine combustion chamber in the presence of thermoacoustic instabilities is based on the proper identification and a thorough understanding of the major mechanisms that drive the dynamics of the parietal transfer. Our objective is to take advantage of our analysis, experimental and computational tools to actively participate in the improvement of the collective knowledge of such kind of transfer. The flow configurations dealt with from the beginning of the project are those of subsonic, single-phase impinging jets or JICF (jets in crossflow) with the possible presence of an interacting acoustic wave. The issue of conjugate heat transfer at the wall will be also gradually investigated. The existing switchover criteria of the hybrid RANS/LES models will be tested on these flow configurations in order to determine their domain of validity. In parallel, the hydrodynamic instability modes of the JICF will be studied experimentally and theoretically (in cooperation with the SIAME laboratory) in order to determine the possibility to drive a change of instability regime (e.g., from absolute to convective) and thus to propose challenging flow conditions that would be relevant for the setting-up of an hybrid LES/DNS approach aimed at supplementing the hybrid RANS/LES approach.
Improvement of turbulence models
The production and subsequent use of DNS (AeroSol library) and experimental (MAVERIC bench) databases dedicated to the improvement of the physical models is a significant part of our activity. In that respect, our present capability of producing in-situ experimental data for simulation validation and flow analysis is clearly a strongly differentiating mark of our project. The analysis of the DNS and experimental data produced make the improvement of the hybrid RANS/LES approach possible. Our hybrid temporal LES (HTLES) method has a decisive advantage over all other hybrid RANS/LES approaches since it relies on a well-defined time-filtering formalism. This feature greatly facilitates the proper extraction from the databases of the various terms appearing in transport equations obtained at the different scales involved (e.g. from RANS to LES). But we would not be comprehensive in that matter if we were not questioning the relevance of any simulation-experiment comparisons. In other words, a central issue is the following question: are we comparing the same quantities between simulations and experiment? From an experimental point of view, the questions to be raised will be, among others, the possible difference in resolution between the experiment and the simulations, the similar location of the measurement points and simulation points, the acceptable level of random error associated to the necessary finite number of samples. In that respect, the recourse to uncertainty quantification techniques will be advantageously considered.
3.2.3 Development of an efficient implicit high-order compressible solver scalable on new architectures
As the flows simulated are very computationally demanding, we will maintain our efforts in the development of AeroSol in the following directions:
- Efficient implementation of the discontinuous Galerkin method.
- Implicit methods based on Jacobian-Free-Newton-Krylov methods and multigrid.
- Porting on heterogeneous architectures.
- Implementation of models.
Efficient implementation of the discontinuous Galerkin method
In high-order discontinuous Galerkin methods, the unknown vector is composed of a concatenation of the unknowns in the cells of the mesh. An explicit residual computation is composed of three loops: an integration loop on the cells, for which computations in two different cells are independent, an integration loop on boundary faces, in which computations depend on data of one cell and on the boundary conditions, and an integration loop on the interior faces, in which computations depend on data of the two neighboring cells. Each of these loops is composed of three steps: the first step consists in interpolating data at the quadrature points; the second step in computing a nonlinear flux at the quadrature points (the physical flux for the cell loop, an upwind flux for interior faces or a flux adapted to the kind of boundary condition for boundary faces); and the third step in projecting the nonlinear flux on the degrees of freedom.
In this research direction, we propose to exploit the strong memory locality of the method (i.e., the fact that all the unknowns of a cell are stocked contiguously). This formulation can reduce the linear steps of the method (interpolation on the quadrature points and projection on the degrees of freedom) to simple matrix-matrix product which can be optimized. For the nonlinear steps, composed of the computation of the physical flux on the cells and of the numerical flux on the faces, we will try to exploit vectorization.
Implicit methods based on Jacobian-Free-Newton-Krylov methods and multigrid
For our computations of the IMPACT-AE project, we have used explicit time stepping. The time stepping is limited by the CFL condition, and in our flow, the time step is limited by the acoustic wave velocity. As the Mach number of the flow we simulated in IMPACT-AE was low, the acoustic time restriction is much lower than the turbulent time scale, which is driven by the velocity of the flow. We hope to have a better efficiency by using time implicit methods, for using a time step driven by the velocity of the flow.
Using implicit time stepping in compressible flows in particularly difficult, because the system is fully nonlinear, such that the nonlinear solving theoretically requires to build many times the Jacobian. Our experience in implicit methods is that the building of a Jacobian is very costly, especially in three dimensions and in a high-order framework, because the optimization of the memory usage is very difficult. That is why we propose to use a Jacobian-free implementation, based on 47. This method consists in solving the linear steps of the Newton method by a Krylov method, which requires Jacobian-vector product. The smart idea of this method is to replace this product by an approximation based on a difference of residual, therefore avoiding any Jacobian computation. Nevertheless, Krylov methods are known to converge slowly, especially for the compressible system when the Mach number is low, because the system is ill-conditioned. In order to precondition, we propose to use an aggregation-based multigrid method, which consists in using the same numerical method on coarser meshes obtained by aggregation of the initial mesh. This choice is driven by the fact that multigrid methods are the only one to scale linearly 63, 64 with the number of unknowns in term of number of operations, and that this preconditioning does not require any Jacobian computation.
Beyond the technical aspects of the multigrid approach, which is challenging to implement, we are also interested in the design of an efficient aggregation. This often means to perform an aggregation based on criteria (anisotropy of the problem, for example) 53. To this aim, we propose to extend the scalar analysis of 65 to a linearized version of the Euler and Navier-Stokes equations, and try to deduce an optimal strategy for anisotropic aggregation, based on the local characteristics of the flow. Note that discontinuous Galerkin methods are particularly well suited to h-p aggregation, as this kind of methods can be defined on any shape 25.
Porting on heterogeneous architectures
Until the beginning of the 2000s, the computing capacities have been improved by interconnecting an increasing number of more and more powerful computing nodes. The computing capacity of each node was increased by improving the clock speed, the number of cores per processor, the introduction of a separate and dedicated memory bus per processor, but also the instruction level parallelism, and the size of the memory cache. Even if the number of transistors kept on growing up, the clock speed improvement has flattened since the mid 2000s 59. Already in 2003, 44 pointed out the difficulties for efficiently using the biggest clusters: "While these super-clusters have theoretical peak performance in the Teraflops range, sustained performance with real applications is far from the peak. Salinas, one of the 2002 Gordon Bell Awards was able to sustain 1.16 Tflops on ASCI White (less than 10% of peak)." From the current multi-core architectures, the trend is now to use many-core accelerators. The idea behind many-core is to use an accelerator composed of a lot of relatively slow and simplified cores for executing the most simple parts of the algorithm. The larger the part of the code executed on the accelerator, the faster the code may become. Therefore, it is necessary to work on the heterogeneous aspects of computations. These heterogeneities are intrinsic to our computations and have two sources. The first one is the use of hybrid meshes, which are necessary for using a locally-structured mesh in a boundary layer. As the different cell shapes (pyramids, hexahedra, prisms and tetrahedra) do not have the same number of degrees of freedom, nor the same number of quadrature points, the execution time on one face or one cell depends on its shape. The second source of heterogeneity are the boundary conditions. Depending on the kind of boundary conditions, user-defined boundary values might be needed, which induces a different computational cost. Heterogeneities are typically what may decrease efficiency in parallel if the workload is not well balanced between the cores. Note that heterogeneities were not dealt with in what we consider as one of the most advanced work on discontinuous Galerkin on GPU 46, as only straight simplicial cell shapes were addressed. For managing at best our heterogeneous computations on heterogeneous architectures, we propose to use the execution runtime StarPU 24. For this, the discontinuous Galerkin algorithm will be reformulated in terms of a graph of tasks. The previous tasks on the memory management will be useful for that. The linear steps of the discontinuous Galerkin methods require also memory transfers, and one issue consists in determining the optimal task granularity for this step, i.e. the number of cells or face integrations to be sent in parallel on the accelerator. On top of that, the question of which device is the most appropriate to tackle such kind of tasks is to be discussed.
Last, we point out that the combination of shared-memory and distributed-memory parallel programming models is better suited than only the distributed-memory one for multigrid, because in a hybrid version, a wider part of the mesh shares the same memory, therefore making a coarser aggregation possible.
These aspects will benefit from a particularly stimulating environment in the INRIA Bordeaux Sud Ouest center around high-performance computing, which is one of the strategic axes of the center.
Implementation of turbulence models in AeroSol and validation
We will gradually insert models developed in research direction 3.2.2 in the AeroSol library in which we develop methods for the DNS of compressible turbulent flows at low Mach number. Indeed, due to its formalism based on temporal filtering, the HTLES approach offers a consistent theoretical framework characterized by a continuous transition from RANS to DNS, even for complex flow configurations (e.g. without directions of spatial homogeneity). As for the discontinuous Galerkin method available presently in AeroSol, it is the best suited and versatile method able to meet the requirements of accuracy, stability and cost related to the local (varying) level of resolution of the turbulent flow at hand, regardless of its complexity. The first step in this direction was taken in 2017 during the internship of Axelle Perraud, who has implemented a turbulence model (--SST) in the Aerosol library.
3.2.4 Validation of the simulations: test flow configurations
To supplement whenever necessary the test flow configuration of MAVERIC and apart from configurations that could emerge in the course of the project, the following configurations for which either experimental data, simulation data or both have been published will be used whenever relevant for benchmarking the quality of our agile computations:
- The impinging turbulent jet (simulations).
- The ORACLES two-channel dump combustor developed in the European projects LES4LPP and MOLECULES.
- The non reactive single-phase PRECCINSTA burner (monophasic swirler), a configuration that has been extensively calculated in particular with the AVBP and Yales2 codes.
- The LEMCOTEC configuration (monophasic swirler + effusion cooling).
- The ONERA MERCATO two-phase injector configuration provided the question of confidentiality of the data is not an obstacle.
- Rotating turbulent flows with wall interaction and heat transfer.
- Turbulent flows with buoyancy.
4 Application domains
4.1 Aeronautics
Cagire is presently involved in studies mainly related to:
- The combustion chamber wall: the modelling, the simulation and the experimentation of the flow around a multiperforated plate representative of a real combustion chamber wall are the three axes we have been developing during the recent period. The continuous improvement of our in-house test facility Maveric is also an important ingredient to produce our own experimental validation data for isothermal flows. For non-isothermal flows, our participation in the EU funded program Soprano will be giving us access to non-isothermal data produced by Onera.
- The flow around airfoils: the modelling of the turbulent boundary layer has been for almost a century a key issue in the aeronautics industry. However, even the more advanced RANS models face difficulties in predicting the influence of pressure gradients on the development of the boundary layer. A main issue is the reliability of the modelling hypotheses, which is crucial for less conservative design. One of the technological barriers is the prediction of the flow in regimes close to the edge of the flight domain (stall, buffeting, unsteady loads) when the boundary layer is slowed down by an adverse pressure gradient. This is the subject of the CIFRE PhD thesis of Gustave Sporschill, started in 2018, in collaboration with Dassault Aviation.
- Impinging jets: because of their high heat transfer efficiency, turbulent impinging jets are commonly used in a large variety of applications, and in particular blade cooling systems. Understanding the underlying physics of the mechanisms at play is of prime interest and is still an open question. Additionally, this configuration remains a challenging test case for turbulence models since it embraces many flow features despite a relatively simple geometry, and causes strong discrepancies between standard turbulence closures. Reynolds stress transport models have been shown to be promising candidates but still suffer from a lack of validation regarding this flow configuration. Such models are the subject of a collaboration with Onera.
- Atmospheric reentry problem: When a body enters the atmosphere with a high velocity, most of its movement is driven by a hypersonic flow surrounding the body. Integrity of the body is maintained by a shield that is progressively ablated. The sharp control of the movement can be driven only provided a very good knowledge of the surrounding hypersonic flow and of the interaction between the ablated shield and the surrounding hypersonic flow. Within the Seigle project, the team is involved in the simulation of the interaction of a droplet (representing the ablated body) and a hypersonic flow. In the Asturies project, the aim is to study the improvement on the shock/turbulence interaction by using higher order RANS models.
4.2 Energy
- The prediction of heat transfer in fluid and solid components is of major importance in power stations, in particular, nuclear power plants. Either for the thermohydraulics of the plenum or in the study of accidental scenarii, among others, the accurate estimation of wall heat transfer, mean temperatures and temperature fluctuations are necessary for the evaluation of relevant thermal and mechanical design criteria. The PhD thesis (CIFRE EDF) of G. Mangeon, defended in February 2020, was dedicated to the development of relevant RANS models for these industrial applications 11.
- Moreover, the prediction of unsteady hydrodynamic loadings is a key point for operating and for safety studies of PWR power plants. Currently, the static loading is correctly predicted by RANS computations but when the flow is transient (as, for instance, in Reactor Coolant Pumps, due to rotor/stator interactions, or during operating transients) or in the presence of large, energetic, coherent structures in the external flow region, the RANS approach is not sufficient, whereas LES is still too costly for a wide use in industry. This issue was the main focus of the PhD thesis (CIFRE EDF) of Vladimir Duffal, defended in November 2020.
- For the design of high temperature solar receiver for concentrated solar power plants, flows are characterized by strong variations of the fluid properties, such that, even in the forced convection regime, they significantly deviate from isothermal flows, with a possible tendency to relaminarize, which can significantly reduce heat transfer. A better understanding and modeling of the physical mechanisms observed in turbulent flows with strong temperature gradients are important and are the focus of a collaboration with the LaTeP laboratory of UPPA 12.
- Thermal storage is interesting to decorrelate the production of heat or cold from its use whether for direct operation as for a heat network (smoothing of heat supply to meet intermittent needs) or for power generation (phase shift between heat generation and power generation). The challenge is to study, via CFD, the dynamic and thermal behavior of the storage during the loading, resting and discharge phases. The PhD thesis of Alexis Ferré, co-supervised by R. Manceau and S. Serra (LaTeP) started in November 2020.
4.3 Automotive propulsion
- The engine (underhood) compartment is a key component of vehicle design, in which the temperature is monitored to ensure the effectiveness and safety of the vehicle, and participates in 5 to 8% of the total drag and CO2 emissions. Dimensioning is an aerodynamic and aerothermal compromise, validated on a succession of road stages at constant speed and stopped phases (red lights, tolls, traffic jam). Although CFD is routinely used for forced convection, state-of-the-art turbulence models are not able to reproduce flows dominated by natural convection during stopped phases, with a Rayleigh number of the order of , such that the design still relies on costly, full-scale, wind tunnel experiments. This technical barrier must be lifted, since the ambition of the PSA group is to reach a full digital design of their vehicles in the 2025 horizon, i.e., to almost entirely rely on CFD. This issue was the focus of the just-defended PhD thesis (CIFRE PSA) of S. Jameel, supervised by R. Manceau, and also a part of the ANR project MONACO_2025 described in section 8.4, in the framework of which S. Jameel will continue working in the team (post-doc, 18 months).
- The Power & Vehicles Division of IFPEN co-develops a CFD code, CONVERGE, to simulate the internal flow in a spark-ignition engine, in order to provide the automotive industry with tools to optimize the design of combustion engines. The RANS method, widely used in the industry, is not sufficiently reliable for quantitative predictions, and is only used as a tool to qualitatively compare different geometries. On the other hand, LES provides more detailed and accurate information, but at the price of a CPU cost unafordable for daily use in the industry. Therefore, IFPEN aims at developing the hybrid RANS/LES methodology, in order to combine the strengths of the two approaches. The PhD thesis of Hassan Afailal, co-supervised by Rémi Manceau, focused on this issue, was defended in December 2020. In the framework of the just-started collaborative project ASTURIES (E2S-UPPA/Inria/CEA/IFPEN), this collaboration with IFPEN will be pursued by the development of high-order methods in the CONVERGE code in order to make it possible to perform highly accurate and low-dissipative LES and hybrid RANS/LES in combustion engines.
5 New software and platforms
5.1 New software
5.1.1 AeroSol
- Keyword: Finite element modelling
- Functional Description: The AeroSol software is a high order finite element library written in C++. The code has been designed so as to allow for efficient computations, with continuous and discontinuous finite elements methods on hybrid and possibly curvilinear meshes. The work of the team CARDAMOM (previously Bacchus) is focused on continuous finite elements methods, while the team Cagire is focused on discontinuous Galerkin methods. However, everything is done for sharing the largest part of code we can. More precisely, classes concerning IO, finite elements, quadrature, geometry, time iteration, linear solver, models and interface with PaMPA are used by both of the teams. This modularity is achieved by mean of template abstraction for keeping good performances. The distribution of the unknowns is made with the software PaMPA , developed within the team TADAAM (and previously in Bacchus) and the team Castor.
-
News of the Year:
In 2020, the following points were addressed in AeroSol
* Update, documentation, and update of wiki for the test case
* Development of Continuous Galerkin based models for Advection, Diffusion and AdvectionDiffusion.
* Implementation of eddy viscosity models (k-epsilon, Spalart-Almarras) turbulence models.
* Keeping on the interfacing with PARMMG for parallel mesh adaptation.
* Development of a HLL flux for finite volume and DG discretizations
* Development of a filtering method for low Mach number flows
* Development of symmetric boundary conditions for Advection and Diffusion problems.
-
URL:
https://
team. inria. fr/ cardamom/ aerosol/ - Authors: Vincent Perrier, Damien Genet, Maxime Mogé, Simon Delmas, Benjamin Lux, Dragan Amenga Mbengoue, Mario Ricchiuto
- Contact: Vincent Perrier
- Participants: Benjamin Lux, Damien Genet, Mario Ricchiuto, Vincent Perrier, Héloïse Beaugendre, Subodh Madhav Joshi, Christopher Poette, Marco Lorini, Jonathan Jung, Enrique Gutierrez Alvarez
- Partner: BRGM
6 New results
6.1 A methodology for simulating unsteady inert or reacting gaseous flows featuring large density variations
Participants: Pascal Bruel, Mariovane Donini.
This work aims at developing an efficient and versatile tool to simulate non-reacting and reacting flow fields in a zero Mach number framework. The formulation and implementation of the unsteady artificial compressibility approach in a rather standard finite volume framework has been carried out in a two-step way. In the first one, only divergence free velocity flow fields were considered, hence imposing quite a severe restriction on the class of reacting flows that could be dealt with. The preliminary results obtained during this first phase have been presented in 13. Then, during the second step, the approach has been generalised so as to deal also with non divergence free velocity flow fields, opening the way for a much broader field of application. The resulting time-accurate scheme was tested in five different cases: the Stokes’ second problem, the unsteady wake flow past a circular cylinder, the flow around a heated cylinder placed in a square enclosure, a steady Tsuji diffusion flame, and a flickering buoyant diffusion flame. The flow over the oscillating plate and past a circular cylinder case were chosen to put into evidence the basic properties of the implemented time-accurate approach, such as the ability to describe unsteady non-reactive flows and its convergence rate. An analysis of the influence of the artificial compressibility factor on the convergence rate was carried out. For the flow in the enclosure hosting a heated cylinder, a configuration that featured quite high density gradients, the predicted flow topology as well as the heat flux distribution along the enclosure walls matched very well those reported in the literature. Then, a comparison with experimental and numerical results was performed for a steady diffusion flame case. The simulated temperature profile and the flame shape were in good agreement with those reported in the literature, except in the region around the maximum temperature, where the reaction layer was not so well described, certainly because of the infinite-rate chemistry assumption considered here. The fifth case investigated the capability of the present approach to predict correctly the large scale unsteadiness of a flickering diffusion flame. An excellent agreement with the experimental results was observed in term of the fundamental frequency of the flame flickering. Qualitatively speaking, the predicted flame topology appeared to be in line with that observed experimentally. A journal paper gathering these results has been submitted.
6.2 Conjugate heat transfer with different fluid-solid physical properties: a new elliptic blending second-moment closure
Participants: Rémi Manceau, Gaëtan Mangeon.
Our approach to model the wall/turbulence interaction, based on Elliptic Blending, was successfully applied to flows with standard thermal boundary conditions at the walls 10. However, Conjugate Heat Transfer, which couples fluid and solid domains, are particularly challenging for turbulence models. We have developed an innovative model, the Elliptic Blending Differential Flux Model, to account for the influence of various wall thermal boundary conditions on the turbulent heat flux and the temperature variance. An assessment of this new model in Conjugate Heat Transfer has been performed for several values of fluid-solid thermal diffusivity and conductivity ratios. A careful attention is paid to the discontinuity of the dissipation rate associated with the temperature variance at the fluid-solid interface. The analysis is supported by successful comparisons with Direct Numerical Simulations. This work led to the publication of an article in Journal of Fluid Mechanics 11. This work constitutes the main part of the thesis of Gaetan Mangeon, defended in 2020 19.
6.3 Development of the EB-RSM model for jets in crossflow, impinging jets and adverse-pressure-gradient boundary layer
Participants: Rémi Manceau, Franck Mastrippolito, Pascal Bruel.
The EB-RSM, developed by the team, is a sophisticated Reynolds-stress model sensitized to the influence of the wall, implemented in several open-source, industrial and commercial codes (OpenFoam, CodeSaturne, AETHER, CEDRE, EZNSS, StarCCM+). Although it has successfully been applied to many different configurations and showed improved results compared to standard industrial models (for instance, 48, 10, 58), some problems have been observed in particular situations, such that the model still requires some improvement.
- In the framework of the SOPRANO European project, it was observed that, when a flow is accelerated inside the holes of a multi-perforated plate, the model is able to predict the laminarization of the flow (not predicted by eddy-viscosity models), but the dissipation does not go properly to zero. This problem has been attributed to the appearance of a pseudo-laminar state 56 and remedied by the introduction of a bound on the turbulent-to-mean time-scale ratio.
- Another aspect concerns the improvement of the prediction of impinging jets, which are known to be wrongly predicted by Reynolds-stress models 62. In collaboration with A. Colombié, F. Chedevergne and E. Laroche from ONERA Toulouse, we have identified by extracting the budgets of the Reynolds stresses in a fine LES computation that this problem is due to the lack of representation of the pressure diffusion mechanisms far from the wall. A model has been proposed for this term that is under validation.
- The CIFRE PhD thesis of Gustave Sporschill (Dassault Aviation) is dedicated to the prediction of the flows around airfoils, and, more generally, boundary layers subject to adverse pressure gradient effects. Different Reynolds-stress models have been evaluated for such flows and showed improved predictions compared to the standard Spalart-Almaras model used routinely by Dassault. Among them, the EB-RSM gives the more accurate results 58 and therefore, is promising as concerns a future application of Reynolds-stress models in the aeronautical sector.
6.4 Eddy-viscosity models sensitized to buoyancy effects for predicting natural convection flows
Participants: Rémi Manceau, Saad Jameel.
Eddy-viscosity turbulence models have been sensitized to the effects of buoyancy, in order to improve the prediction in natural convection flows. The approach extends in a linear way the constitutive relations for the Reynolds stress and the turbulent heat flux, in order to account for the anisotropic influence of buoyancy. The novelty of this work involves the buoyancy extension applied to three very different eddy-viscosity models, which leads to encouraging results for the highly challenging case of the differentially heated vertical channel and the differentially heated cavity 42, 43. This models were developed during the PhD of Saad Jameel, defended in December 2020 18.
In the framework of the ANR MONACO project (post-doc of Saad Jameel), in collaboration with PSA Group, these models are implemented in the commercial code ANSYS Fluent and applied to real underhood configurations of vehicules in situations driven by natural convection.
6.5 Development of the HTLES approach
Participants: Rémi Manceau, Vladimir Duffal, Hassan Afailal, Franck Mastrippolito, Pascal Bruel, Puneeth Bikkanahally.
The HTLES (hybrid temporal LES) approach, developed by the team, has been improved by introducing shielding functions and an internal consistency constraint to enforce the RANS behavior in the near-wall regions 51. The influence of the underlying closure model was studied by applying HTLES to two RANS models: the k- SST and BL-v2/k models. The validation, carried out using two different solvers, Code_Saturne (collaboration with EDF) and Converge-CFD (collaboration with IFPEN), encompassed different type of internal flows: channel, periodic hill, internal combustion engines (in fixed position and with a moving piston), jet in crossflow 36, 21. The robustness of HTLES to grid coarsening and the accuracy of the predictions at a reduced numerical cost compared to LES was demonstrated. Notably, the capacity of HTLES to provide information on velocity and pressure temporal fluctuations at the wall was assessed, offering a cost-saving alternative to LES to predict unsteady loads. One of the major limitation of HTLES, i.e., the fact that it is based on statistically averaged quantities, which are challenging to estimate in non-stationary flows, has been removed, by approximating the statistical average by a Dynamic Temporal Filter. These developments were carried out in the framework of the European project SOPRANO; and during the PhD thesis of Vladimir Duffal (part of the ANR project MONACO), defended in November 2020 17; and during the PhD thesis of Al Hassan Afailal, defended in December 2020 16.
In the framework of the ANR project MONACO, the HTLES approach is extended to flows influenced by buoyancy. During the PhD thesis of Puneeth Bikkanahally, the model has been applied to cavity flows, and in particular the strongly stratified cavity of 57. Due to the co-existence of a turbulent, buoyant boundary layer and a laminar region in the center of the cavity, the shielding function based on the Kolmogorov scale developed during the thesis of Vladimir Duffal 16 deteriorates the results. The ongoing work is dedicated to the development of an alternative shielding function based on the resolution of an elliptic equation 29.
6.6 Compact WENO, positivity preserving stabilization of discontinuous Galerkin methods
Participants: Vincent Perrier.
Jointly with Alireza Mazaheri (NASA Langley) and Chi Wang Shu, we have developed a compact WENO stabilization that moreover ensures the positivity of physical quantities. The work was published in 52.
7 Bilateral contracts and grants with industry
7.1 Bilateral contracts with industry
- EDF: "Advanced modelling of heat transfer for industrial configurations with or without accounting of the solid wall", contract associated to the PhD thesis of Gaëtan Mangeon
- EDF: "Hybrid RANS/LES modelling for unsteady loadings in turbulent flows", contract associated to the PhD thesis of Vladimir Duffal
- IFPEN: "3D simulation of non-reactive internal aerodynamics of spark-ignition engines using an hybrid RANS/LES method", contract associated to the PhD thesis of Hassan Al Afailal
- PSA: ""Turbulence modelling in the mixed and natural convection regimes in the context of automotive applications", contract associated to the PhD thesis of Saad Jameel.
- Dassault Aviation: "Improvement of the turbulence models”, contract associated to the PhD thesis of Gustave Sporschill.
- CEA: “Agile simulation of turbulent internal flows”, contract in the framework of the Asturies project.
7.2 Bilateral grants with industry
- EDF (Cifre PhD grant): "Advanced modelling of heat transfer for industrial configurations with or without accounting of the solid wall", PhD student: Gaëtan Mangeon
- EDF (Cifre PhD grant): "Hybrid RANS/LES modelling for unsteady loadings in turbulent flows", PhD student: Vladimir Duffal
- IFPEN (PhD grant): "3D simulation of non-reactive internal aerodynamics of spark-ignition engines using an hybrid RANS/LES method", PhD sutdent: Hassan Al Afailal
- PSA (Cifre PhD grant): "Turbulence modelling in the mixed and natural convection regimes in the context of automotive applications", PhD student: Saad Jameel.
- Dassault Aviation (Cifre PhD grant): "Improvement of the turbulence models. Application to the prediction of aerodynamic flows", PhD student: Gustave Sporschill.
- CEA: “CFD and experimental study of a thermocline-type thermal storage for an optimized design and data entry of component scale models in the framework of a multi-scale approach”, PhD student Alexis Ferré.
- CEA: “Development of Fast, Robust and Accurate numerical methods for turbulence models on Complex Meshes” (1/2 Grant), PhD student, Anthony Bosco.
8 Partnerships and cooperations
8.1 International initiatives
8.1.1 Participation in other international programs
-
National University of Córdoba (UNC), Córdoba, Argentina: ECOS-Sud A17A07 project
Participants: Pascal Bruel.
2020 was the third and last year of this project devoted to the simulations of the wind around aerial fuel tanks and related experiments. All planned stays for 2020 have been cancelled, severly impacting the final course of the program. The main activity was directed towards the formatting of a database incorporating the velocity data collected during the test campaign of September 2019 during which PIV measurements of the velocity fields of flows around two wall mounted cylinders in a tandem configuration were carried out.
8.2 International research visitors
8.2.1 Visits of international scientists
During 2020, Mariovane Sabino Donini (Instituto Nacional de Pesquisas Espaciais - (INPE) - Brazil) spent the last eight months of his 12-month stay in the team. He worked with P. Bruel to develop a numerical methodology to simulate inert and reacting gaseous flows featuring large density variations. This is the first time that the simulation of reacting flows (diffusion flames in that case) is considered in the team. As a first step, a Boussinesq-like approximation was adopted do deal with these reacting flows and a first version of the program was developed on that basis. As expected, for reacting flows, the validation test cases carried out clearly evidenced the limitations of such an approximation as soon as the Froude number is sufficiently small to induce a strong buoyancy related flame unsteadiness 13. Thus, the methodology has been adapted to remove this limitations and the first results obtained with the new version of the code proved to be quite promising.
8.3 European initiatives
8.3.1 FP7 & H2020 Projects
SOPRANO
Participants: Pascal Bruel, Rémi Manceau, Franck Mastrippolito.
- Topic: MG-1.2-2015 - Enhancing resource efficiency of aviation
- Project acronym: SOPRANO
- Project title: Soot Processes and Radiation in Aeronautical inNOvative combustors
- Duration: 01/09/2016 - 28/02/2021
- Coordinator: SAFRAN
- Other partners:
- France: CNRS, CERFACS, INSA Rouen, SAFRAN SA, Snecma SAS, Turbomeca SA.
- Germany: DLR, GE-DE Gmbh, KIT, MTU, RRD,
- Italy: GE AVIO SRL, University of Florence
- United Kingdom: Rolls Royce PLC, Imperial College of Science, Technology and Medecine, Loughborough University.
- Abstract:
For decades, most of the aviation research activities have been focused on the reduction of noise and NOx and
CO2 emissions. However, emissions from aircraft gas turbine engines of non-volatile PM, consisting primarily of
soot particles, are of international concern today. Despite the lack of knowledge toward soot formation processes
and characterization in terms of mass and size, engine manufacturers have now to deal with both gas and particles
emissions. Furthermore, heat transfer understanding, that is also influenced by soot radiation, is an important matter
for the improvement of the combustor's durability, as the key point when dealing with low-emissions combustor
architectures is to adjust the air flow split between the injection system and the combustor's walls. The SOPRANO
initiative consequently aims at providing new elements of knowledge, analysis and improved design tools, opening the
way to:
- Alternative designs of combustion systems for future aircrafts that will enter into service after 2025 capable of simultaneously reducing gaseous pollutants and particles,
- Improved liner lifetime assessment methods. Therefore, the SOPRANO project will deliver more accurate experimental and numerical methodologies for predicting the soot emissions in academic or semi-technical combustion systems. This will contribute to enhance the comprehension of soot particles formation and their impact on heat transfer through radiation. In parallel, the durability of cooling liner materials, related to the walls air flow rate, will be addressed by heat transfer measurements and predictions. Finally, the expected contribution of SOPRANO is to apply these developments in order to determine the main promising concepts, in the framework of current low-NOx technologies, able to control the emitted soot particles in terms of mass and size over a large range of operating conditions without compromising combustor's liner durability and performance toward NOx emissions.
- In the SOPRANO project, our objective is to complement the experimental (ONERA)
and LES (CERFACS) work by RANS computations of the flow around a multiperforated plate.
Franck Mastrippolito, the post-doc recruited from mid-january 2019 to mid-january 2020, performed simulations aimed at reproducing the experiment of ONERA Toulouse carried out in the same workpackage. The configuration is that of an effusion plate with a gyration angle of 90 degrees and the turbulence model is EBRSM.
Figure 2: Simulation of the ONERA SOPRANO configuration: example of experimental (top) vs numerical (bottom) results concerning the mean velocity field.
8.4 National initiatives
GIS Success
Participants: Pascal Bruel.
We are member of the CNRS GIS Success (Groupement d'Intérêt Scientifique) organised around two of the major CFD codes employed by the Safran group, namely AVBP and Yales2. This year, the evaluation of the capability of the compressible module of Yales2 at low Mach number has been carried out.ANR MONACO_2025
Participants: Rémi Manceau.
The ambition of the MONACO_2025 project, coordinated by Rémi Manceau, is to join the efforts made in two different industrial sectors in order to tackle the industrial simulation of transient, turbulent flows affected by buoyancy effects. It brings together two academic partners, the project-team Cagire hosted by the university of Pau, and the institute Pprime of the CNRS/ENSMA/university of Poitiers (PPRIME), and R&D departments of two industrial partners, the PSA group and the EDF group, who are major players of the automobile and energy production sectors, respectively.
- The main scientific objective of the project is to make a breakthrough in the unresolved issue of the modelling of turbulence/buoyancy interactions in transient situations, within the continuous hybrid RANS/LES paradigm, which consists in preserving a computational cost compatible with industrial needs by relying on statistical approaches where a fine-grained description of the turbulent dynamics is not necessary. The transient cavity flow experiments acquired during MONACO_2025 will provide the partners and the scientific community with an unrivalled source of knowledge of the physical mechanisms that must be accounted for in turbulence models.
- The main industrial objective is to make available computational methodologies to address dimensioning, reliability and security issues in buoyancy-affected transient flows. It is to be emphasized that such problems are not tackled using CFD at present in the industry. At the end of MONACO_2025, a panel of methodologies, ranging from simple URANS to sophisticated hybrid model based on improved RANS models, will be evaluated in transient situations, against the dedicated cavity flow experiments and a real car underhood configuration. This final benchmark exercise will form a decision-making tool for the industrial partners, and will thus pave the way towards high-performance design of low-emission vehicles and highly secure power plants. In particular, the project is in line with the Full Digital 2025 ambition, e.g., the declared ambition of the PSA group to migrate, within the next decade, to a design cycle of new vehicles nearly entirely based on CAE (computer aided engineering), without recourse to expensive full-scale experiments.
8.5 Regional initiatives
8.5.1 SEIGLE
Participants: Enrique Gutierrez Alvarez, Jonathan Jung, Vincent Perrier.
SEIGLE means "Simulation Expérimentation pour l'Interaction de Gouttes Liquides avec un Ecoulement fortement compressible". It is a 3-year program which has started since October 2017 and was funded by Région Nouvelle-Aquitaine, ISAE-ENSMA, CESTA and Inria. The interest of understanding aerodynamic mechanisms and liquid drops atomization is explained by the field of applications where they play a key role, specially in the new propulsion technologies through detonation in the aerospace as well as in the securities field. The SEIGLE project was articulated around a triptych experimentation, modeling and simulation. An experimental database will be constituted. It will rely on a newly installed facility (Pprime), similar to a supersonic gust wind tunnel/ hypersonic from a gaseous detonation tube at high pressure. This will allow to test modeling approaches (Pprime / CEA) and numerical simulation (Inria / CEA) with high order schemes for multiphasic compressible flows, suitable for processing shock waves in two-phase media.
8.5.2 HPC scalable ecosystem
Participants: Jonathan Jung, Vincent Perrier, Sangeeth Simon.
HPC scalable ecosystem is a 3-year program funded by Région Nouvelle-Aquitaine (call 2018), Airbus, CEA-CESTA, University of Bordeaux, INRA, ISAE-ENSMA and Inria. A two-year post-doc will be hired in 2019 or 2020. The objective is to extend the prototype developed in 37 to high order (discontinuous Galerkin) and non-reactive diffusive flows in 3d. The same basis will be developed in collaboration with Pprime for WENO based methods for reactive flows.
9 Dissemination
9.1 Promoting scientific activities
9.1.1 Journal
Member of the editorial boards
- Visualization of Mechanical Processes [Pascal Bruel]
- Advisory Board of International Journal of Heat and Fluid Flow [Rémi Manceau]
- Advisory Board of Flow, Turbulence and Combustion [Rémi Manceau]
Reviewer - reviewing activities
- Phys. Rev. Fluids [Rémi Manceau]
- Energies [Rémi Manceau]
- Computer & Fluids [Rémi Manceau,Jonathan Jung]
- Journal of Computational Physics [Jonathan Jung]
- Aerospace Science and Technology [Pascal Bruel]
- Revista Facultad de Ingeniería Universidad de Antioquia [Pascal Bruel]
9.1.2 Leadership within the scientific community
- Rémi Manceau co-organizes the activities of the Special Interest Group 15 (Turbulence modelling) of ERCOFTAC (European Research Community on Flow, Turbulence and Combustion) as a member of the Steering Committee.
- Rémi Manceau coordinates the ANR Project MONACO_2025, a 4-year project started in 2018. The partners are: the institute PPrime, PSA Group and EDF.
9.1.3 Research administration
- Co-responsible for the organisation of the LMAP seminar of Mathematics and their Applications [Jonathan Jung].
- Member of the LMAP council [Jonathan Jung, Pascal Bruel].
- Member of the IPRA research federation scientific council [Rémi Manceau].
- Member of the CUMI-R [Vincent Perrier].
- Member of the CDT, in charge of the evaluation of software projects at the Inria Bordeaux center [Vincent Perrier].
- Elected member of the Inria evaluation committee, and member of the board [Vincent Perrier]. 8
- Member of the CT3-Num committee of Pau University, in charge of managing the computing resources and projects at Pau University [Vincent Perrier].
9.2 Teaching - Supervision - Juries
9.2.1 Teaching
- In charge second year license of the Mathematics-Computer Science [Jonathan Jung]
- In charge of the second year of the cursus Master in Engineering speciality Mathematics and Computer Science [Jonathan Jung]
- In charge of the third year of the cursus Master in Engineering speciality Mathematics and Computer Science [Jonathan Jung]
- In charge of the fourth year of the cursus Master in Engineering speciality Mathematics and Computer Science [Jonathan Jung]
- In charge of the fifth year of the cursus Master in Engineering speciality Mathematics and Computer Science [Jonathan Jung]
- License : [Jonathan Jung], Descriptive statistical, 22.5h, L1 - MIASHS, Université de Pau et des Pays de l'Adour, Pau, France.
- License : [Jonathan Jung], Research and innovation, 1.5h, L1, Université de Pau et des Pays de l'Adour, Pau, France.
- License : [Jonathan Jung], Numerical analysis for vectorial problems, 42.75h, L2 - Mathematics, Université de Pau et des Pays de l'Adour, Pau, France.
- Master : [Jonathan Jung], Data analysis, 68h25, M1 - GP, Université de Pau et des Pays de l'Adour, Pau, France.
- Master : [Jonathan Jung], Tools for scientific computing, 48h75, M1 - MMS, Université de Pau et des Pays de l'Adour, Pau, France.
- Master : [Jonathan Jung], Supervised personal work, 5 h, M1 - MMS, Université de Pau et des Pays de l'Adour, Pau, France.
- Master: [Vincent Perrier], Numerical analysis of PDE 1, Master MMS, Pau.
- Master : “Turbulence modelling” (in English), 27h30, M2 - International Master program Turbulence, Université de Poitiers/Ecole centrale de Lille, France. [Rémi Manceau]
- Eng. 3 : “Industrial codes for CFD” (in English), 12h30, 3rd year of engineering school (M2), ENSMA, Poitiers, France. [Rémi Manceau]
- Eng. 3 : “Advanced physics–Turbulence modelling for CFD”, 16h, 3rd year of engineering school (M2), ENSGTI, France. [Rémi Manceau]
9.2.2 Supervision
- PhD in progress: Puneeth Bikkanahally Muni Reddy, "Modelling turbulent flows in natural convection regimes using hybrid RANS-LES approaches, UPPA, October 2018, Rémi Manceau.
- PhD, defended in 2020: Gaëtan Mangeon, "Advanced modelling of heat transfer for industrial configurations with or without accounting of the solid wall", UPPA, February 2017, Rémi Manceau.
- PhD, defended in 2020: Vladimir Duffal, "Hybrid RANS/LES modelling for unsteady loadings in turbulent flows", UPPA, November 2017, Rémi Manceau.
- PhD, defended in 2020: Hassan Al Afailal: "3D simulation of non-reactive internal aerodynamics of spark-ignition engines using an hybrid RANS/LES method", September 2017, Rémi Manceau.
- PhD, defended in 2020: Saad Jameel: "Turbulence modelling in the mixed and natural convection regimes in the context of automotive applications", UPPA, February 2017, Rémi Manceau.
- PhD in progress: Gustave Sporschill, "Amélioration des modèles pour la turbulence. Applications à la prédiction des écoulements aérodynamiques", UPPA, May 2018, Rémi Manceau.
- PhD in progress: Mahitosh Mehta, “Development of an agile methodology for hybrid RANS-LES computations of turbulent flows”, UPPA, E2S-UPPA Asturies project, 1/11/20, Rémi Manceau
- PhD in progress: Alexis Ferré, “CFD and experimental study of a thermocline-type thermal storage for an optimized design and data entry of component scale models in the framework of a multi-scale approach”, CEA LITEN, 1/11/20, Rémi Manceau
- PhD in progress: Anthony Bosco, “Development of Fast, Robust and Accurate numerical methods for turbulence models on Complex Meshes” CEA/E2S-UPPA, 1/1/21, Vincent Perrier and Jonathan Jung.
9.2.3 Juries
The participation in the following thesis juries is noted:
- Referee/Opponent, Philippe NGuyen, University of Manchester [Rémi Manceau]
- Referee, Damien Toussaint, Institut National Polytechnique de Toulouse [Rémi Manceau]
- Referee, Gaëtan Crouzy, Université de Toulouse [Pascal Bruel]
The participation in the following HDR jury is noted:
- Referee/President, Olivier Soulard, Université Claude Bernard Lyon 1 / École centrale de Lyon [Pascal Bruel]
9.3 Popularization
9.3.1 Interventions
- R. Manceau. Mon écoulement est turbulent, c'est grave docteur ? Les enjeux de la simulation numérique en mécanique des fluides. Unithéoucafé. 20
- P. Bruel participated in the «Forum des Métiers» organized by Collège Pierre Emmanuel (Pau (64), France) on February 21, 2020. He manned a booth during the all day with the objective of explaining the activity of researcher to an audience of middle school students.
10 Scientific production
10.1 Major publications
- 1 articleA low Mach correction able to deal with low Mach acousticsJournal of Computational Physics3782019, 723--759
- 2 article Construction of modified Godunov type schemes accurate at any Mach number for the compressible Euler system Mathematical Models and Methods in Applied Sciences November 2016
- 3 articleLES fluid-solid coupled calculations for the assessment of heat transfer coefficient correlations over multi-perforated wallsAerospace Science and Technology532016, 13
- 4 articleRunge-Kutta discontinuous Galerkin method for the approximation of Baer and Nunziato type multiphase modelsJournal of Computational Physics23111February 2012, 4096-4141
- 5 articleToward an equivalence criterion for Hybrid RANS/LES methodsComputers and Fluids1222015, 233-246
- 6 article An interface condition to compute compressible flows in variable cross section ducts Comptes Rendus Mathématiques February 2016
- 7 articleRecent progress in the development of the Elliptic Blending Reynolds-stress modelInt. J. Heat Fluid Fl.512015, 195-220URL: http://dx.doi.org/10.1016/j.ijheatfluidflow.2014.09.002
- 8 articleExtension to various thermal boundary conditions of the elliptic blending model for the turbulent heat flux and the temperature varianceJournal of Fluid Mechanics905A1December 2020, 1-34
- 9 articleGodunov-type schemes with an inertia term for unsteady full Mach number range flow calculationsJournal of Computational Physics281January 2015, 35
10.2 Publications of the year
International journals
International peer-reviewed conferences
Conferences without proceedings
Doctoral dissertations and habilitation theses
10.3 Other
Scientific popularization
10.4 Cited publications
- 21 articleDevelopment and validation of a hybrid temporal LES model in the perspective of applications to internal combustion enginesOil & Gas Science and Technology - Revue d'IFP Energies nouvelles742019, 56
- 22 articleAn interior penalty finite element method with discontinuous elementsSIAM journal on numerical analysis1941982, 742--760
- 23 articleUnified analysis of discontinuous Galerkin methods for elliptic problemsSIAM journal on numerical analysis3952002, 1749--1779
- 24 articleStarPU: A Unified Platform for Task Scheduling on Heterogeneous Multicore ArchitecturesConcurr. Comput. : Pract. Exper.232February 2011, 187--198URL: http://dx.doi.org/10.1002/cpe.1631
- 25 articleOn the flexibility of agglomeration based physical space discontinuous Galerkin discretizationsJournal of Computational Physics23112012, 45 - 65URL: http://www.sciencedirect.com/science/article/pii/S0021999111005055
- 26 articleDiscontinuous Galerkin solution of the Reynolds-averaged Navier-Stokes and k-omega turbulence model equationsComputers & Fluids344-52005, 507-540
- 27 articleA high-order accurate discontinuous finite element method for the numerical solution of the compressible Navier-Stokes equationsJ. Comput. Phys.13121997, 267--279URL: http://dx.doi.org/10.1006/jcph.1996.5572
- 28 inproceedingsA high-order accurate discontinuous finite element method for inviscid and viscous turbomachinery flowsProceedings of the 2nd European Conference on Turbomachinery Fluid Dynamics and ThermodynamicsTechnologisch Instituut, Antwerpen, Belgium1997, 99--109
- 29 conference Development of a hybrid RANS-LES model based on temporal filtering for natural convection flows 14th World Congress Comput. Mech. (WCCM), ECCOMAS Congress 2020, Paris (Virtual Congress), France 2021
- 30 articleThe Runge-Kutta local projection discontinuous Galerkin finite element method for conservation laws. IV. The multidimensional caseMath. Comp.541901990, 545--581URL: http://dx.doi.org/10.2307/2008501
- 31 articleTVB Runge-Kutta local projection discontinuous Galerkin finite element method for conservation laws. III. One-dimensional systemsJ. Comput. Phys.8411989, 90--113
- 32 articleTVB Runge-Kutta local projection discontinuous Galerkin finite element method for conservation laws. II. General frameworkMath. Comp.521861989, 411--435URL: http://dx.doi.org/10.2307/2008474
- 33 articleThe Runge-Kutta discontinuous Galerkin method for conservation laws. V. Multidimensional systemsJ. Comput. Phys.14121998, 199--224URL: http://dx.doi.org/10.1006/jcph.1998.5892
-
34
articleThe Runge-Kutta local projection
-discontinuous-Galerkin finite element method for scalar conservation laws'RAIRO Modél. Math. Anal. Numér.2531991, 337--361 - 35 inproceedings Discontinuous Galerkin methods for turbulence simulation Proceedings of the Summer Program Center for Turbulence Research 2002
- 36 inproceedings Development and validation of a hybrid RANS/LES approach based on temporal filtering ASME - JSME - KSME Joint Fluids Engineering Conference 2019 Proc. ASME - JSME - KSME Joint Fluids Engineering Conference 2019, Jul 2019, San Francisco, CA, United States. San Francisco, CA, United States July 2019
- 37 articleA task-driven implementation of a simple numerical solver for hyperbolic conservation lawsESAIM: Proceedings and Surveys63January 2017, 228-247
- 38 articleOn a robust discontinuous Galerkin technique for the solution of compressible flowJ. Comput. Phys.22412007, 208--221URL: http://dx.doi.org/10.1016/j.jcp.2007.01.035
- 39 book Turbulence: The Legacy of AN Kolmogorov Cambridge University Press 1995
- 40 article Non-Reflecting Boundary Conditions for Euler Equation Calculation The American Institute of Aeronautics and Astronautics Journal 42 12 1990
- 41 articleSymmetric interior penalty DG methods for the compressible Navier-Stokes equations. I. Method formulationInt. J. Numer. Anal. Model.312006, 1--20
- 42 inproceedings A buoyancy extension for eddy-viscosity models for the natural convection regime 17th European Turbulence Conference (ETC-2019) Torino, Italy September 2019
- 43 inproceedings Sensitization of eddy-viscosity models to buoyancy effects for predicting natural convection flows HEFAT 2019 - 14th International Conference on Heat Transfer, Fluid Mechanics and Thermodynamics Proc. HEFAT 2019 - 14th International Conference on Heat Transfer, Fluid Mechanics and Thermodynamics Wicklow, Ireland July 2019
- 44 inproceedings Using Computational Fluid Dynamics for Aerodynamics National Research Council Workshop on "The Future of Supercomputing" 2003
- 45 articleOn the convergence of shock-capturing streamline diffusion finite element methods for hyperbolic conservation lawsMath. Comp.541891990, 107--129URL: http://dx.doi.org/10.2307/2008684
- 46 articleNodal discontinuous Galerkin methods on graphics processorsJournal of Computational Physics228212009, 7863 - 7882URL: http://www.sciencedirect.com/science/article/pii/S0021999109003647
- 47 articleJacobian-free Newton-Krylov methods: a survey of approaches and applicationsJournal of Computational Physics19322004, 357 - 397URL: http://www.sciencedirect.com/science/article/pii/S0021999103004340
- 48 inproceedings Computations of complex flow configurations using a modified Elliptic-Blending Reynolds-Stress model ETMM10 2014
- 49 incollectionOn a finite element method for solving the neutron transport equationMathematical aspects of finite elements in partial differential equations (Proc. Sympos., Math. Res. Center, Univ. Wisconsin, Madison, Wis., 1974)Math. Res. Center, Univ. of Wisconsin-Madison, Academic Press, New York1974, 89--123. Publication No. 33
- 50 articleAn explicit discontinuous Galerkin scheme with local time-stepping for general unsteady diffusion equationsJ. Comput. Phys.227112008, 5649--5670URL: http://dx.doi.org/10.1016/j.jcp.2008.02.015
- 51 articleHybrid temporal LES: Development and applicationsERCOFTAC Bulletin1202019, 38-42
- 52 articleBounded and compact weighted essentially nonoscillatory limiters for discontinuous Galerkin schemes: Triangular elementsJournal of Computational Physics395October 2019, 461-488
- 53 articleAnalysis of Aggregation-Based MultigridSIAM J. Sci. Comput.302March 2008, 1082--1103URL: http://dx.doi.org/10.1137/060678397
- 54 articleBoundary conditions for direct simulations of compressible viscous flowsJournal of Computational Physics1011992, 104-129
- 55 techreport Triangular mesh methods for the neutron transport equation LA-UR-73-479 Los Alamos Scientific Laboratory 1973
- 56 articleArbitrary steady-state solutions with the $k$-$\varepsilon$ modelAIAA J.442006, 1586-1592
- 57 articleDirect numerical simulation of an air-filled differentially heated square cavity with Rayleigh numbers up to $10^{11}$Int. J. Heat Mass Tran.1232018, 297-319
- 58 conference Reynolds stress RANS models for industrial aeronautical applications 14th World Congress Comput. Mech. (WCCM), ECCOMAS Congress 2020, Paris (Virtual Congress), France 2021
- 59 article The free lunch is over: A fundamental turn toward concurrency in software Dr. Dobb's Journal 2005
- 60 articleTime-dependent boundary conditions for hyperbolic systems, \II\Journal of Computational Physics8921990, 439 - 461URL: http://www.sciencedirect.com/science/article/pii/002199919090152Q
- 61 articleArtificial boundary conditions for the numerical solution of the Euler equations by the discontinuous galerkin methodJournal of Computational Physics230152011, 5974 - 5995URL: http://www.sciencedirect.com/science/article/pii/S0021999111002324
- 62 phdthesis Lois de paroi adaptatives pour un modèle de fermeture du second ordre dans un contexte industriel Université de Pau et des Pays de l'Adour May 2016
- 63 bookAn introduction to multigrid methodsPure and applied mathematicsChichester, New YorkJ. Wiley1992, URL: http://opac.inria.fr/record=b1088946
- 64 articleWhy Multigrid Methods Are So EfficientComputing in Science and Engg.86November 2006, 12--22URL: http://dx.doi.org/10.1109/MCSE.2006.125
- 65 articlehp-Multigrid as Smoother algorithm for higher order discontinuous Galerkin discretizations of advection dominated flows: Part I. Multilevel analysisJournal of Computational Physics231222012, 7537 - 7563URL: http://www.sciencedirect.com/science/article/pii/S0021999112003129